What Stars Are Visible at Different Times of the Year?
As Earth orbits the Sun, the apparent position of the Sun against the background stars changes. As a result, we see different parts of the sky at different times of the year. This annual motion of Earth around the Sun is what gives us our “winter sky” and our “summer sky,” respectively.
Apparent Motion and Actual Motion
You are probably aware that Earth orbits the Sun once every year. But have you thought about what that means for the stars we see in the sky at night? In this post we will look at how Earth’s motion affects the stars we can see in the night sky.
First, we have to be clear about one thing: The actual motion of the stars through space is so small that it is completely imperceptible to us. When we observe over the course of a year, the stars’ motion is so slight, at least if we use just our eyes, that their relation to one another appears completely constant. If we use special equipment over a long enough time, then we can measure the motions of some stars. But we cannot observe the motion of the stars with naked eye observations, even over a period of many years. Thus, any motion we observe in the stars is due completely to Earth’s motion.
One example of this observed stellar motion is caused by Earth’s rotation about its spin axis. As a result of Earth spinning, the stars appear to circle around the Earth every day. This daily (or diurnal) motion makes the stars - including the Sun - seem to rise in the east and set in the west.
Apparent motion of the stars can also be due to Earth’s orbital motion around the Sun. This type of motion is called annual motion because it happens over the entire year, not just over one day. In truth, both effects contribute to how things appear to move for an Earth-bound observer.
Unlike the stars, there are a few things that really do move in perceptible ways, even leaving aside the motion of Earth itself. These are the Moon and planets. The Moon orbits Earth, and the planets orbit the Sun. You probably know that the moon requires one month to travel one time around Earth. That is where the word “month” comes from. Sounds a little bit like “moon,” doesn’t it? The orbital period for each planet to revolve around the Sun is unique, and the farther out a planet lies, the longer it takes. So the apparent motions of these objects is some combination of their innate motion superimposed upon their apparent motion caused by the motion of Earth. We won’t worry about the planets anymore in this post, but we wanted to mention them so as to avoid confusion regarding their peculiar motions in the sky. From here onward we will be concerned only with the apparent motion of the stars, including the Sun
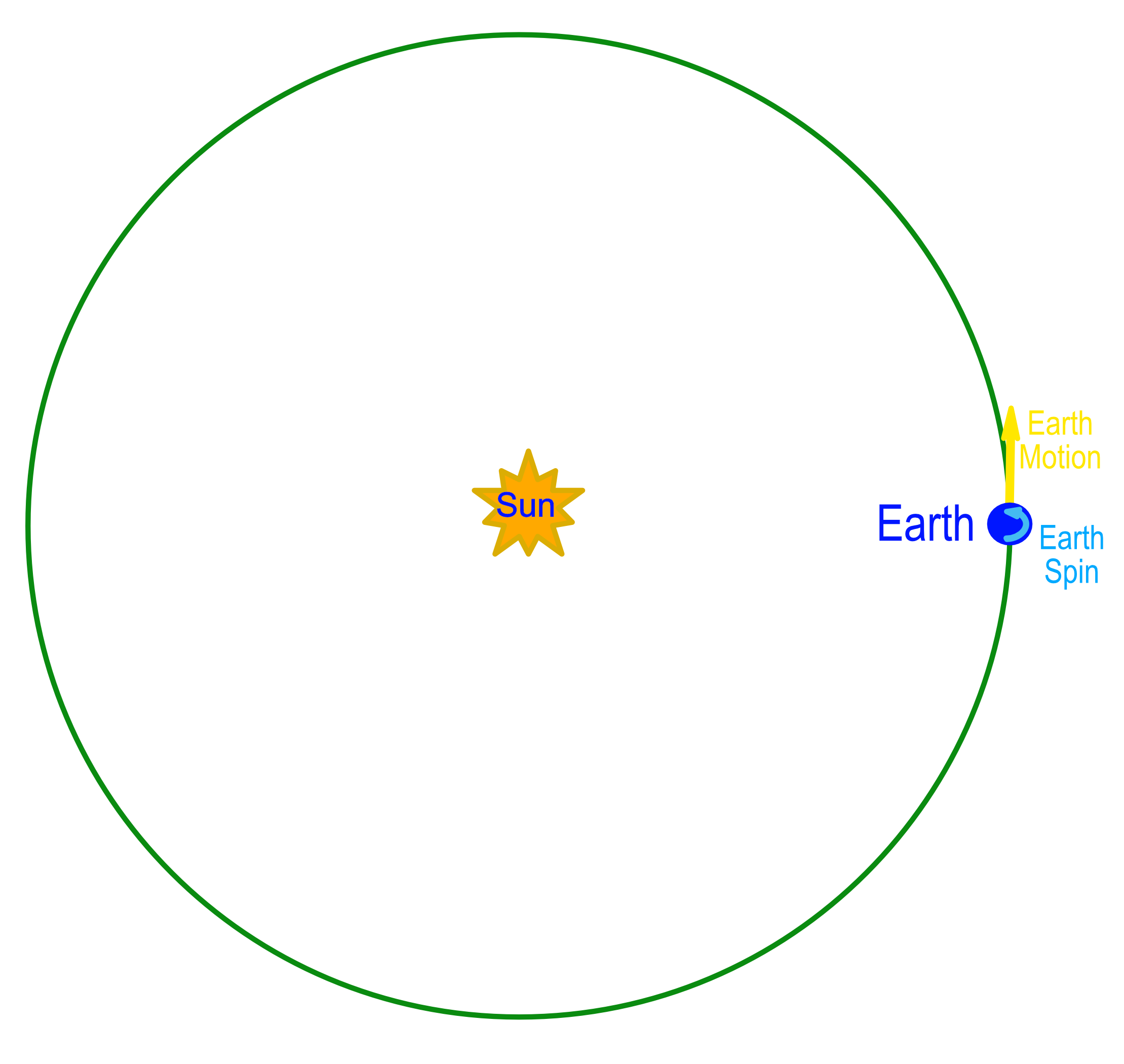
Have a look at the image above. It shows a view of Earths orbit around the Sun from a point located directly above the Sun. The orbit lies in a plane (the plane of your screen in this diagram) called the ecliptic plane, or simply the ecliptic. To have this perspective, we are on a line perpendicular to the ecliptic and passing through the Sun. We will assume our location is in the direction generally above Earth’s Northern Hemisphere. We are not exactly in the direction of the North Pole because the spin axis is tilted about 23 degrees with respect to the ecliptic. From our vantage point, Earth moves counterclockwise around the Sun. This direction is indicated by the yellow arrow. The orbital path of Earth is represented by the green circle; the actual orbit is not exactly circular, but it is very, very close.
The spin direction of Earth is also indicated, by the light blue arrow superimposed on the disk of Earth. Place your right hand in a fist with your pinking touching the screen. Extend your thumb upward away from the screen. Your thumb indicates the (very approximate) direction of the North Pole of the spin axis, neglecting the aforementioned 23 degree tilt. Your fingers curl around in the direction of Earth’s rotation; just as its revolution about the Sun, Earth’s rotation is counterclockwise when viewed from above the Northern Hemisphere. (Note that we will generally use revolution to indicate orbital motion through space. Rotation will be used to indicate spin about an axis.)
Keep in mind that this and the other diagrams here are nowhere near to scale. If we showed the Sun and Earth on the same linear scale as Earth’s orbit they would both be tiny dots. We want to show some details that would not be visible on a scale diagram, so remember as you go through this posting that the diagrams are schematic and are intended to illustrate the ideas we discuss. They should not be taken as realistic or literal representations of the solar system.
The image below shows the Earth-Sun system from an oblique perspective. We are still above the ecliptic, but now we are not directly above the Sun, we are off to the side and outside Earth’s orbit. From this perspective you can more easily see the tilt of Earth’s spin axis, indicated by the red arrows. Don’t be confused by the shape of Earth’s orbit in this view. The orbit is still nearly circular, but we are viewing it at an oblique angle that foreshortens it along our line of sight.
You can experience this same effect if you hold a coin and slowly rotate it so that it is seen first edge-on and then face-on. In between, the angle will make the circular coin appear elliptical (in projection), just as Earth’s orbit appears elliptical in the diagram below.
The diagram shows Earth in four different positions in its orbit. These correspond to the four different months shown. Note that the spin axis, indicated by the red arrows, always points toward the same direction in the sky. It never changes its orientation as Earth orbits the Sun. The arrow points toward north in the diagram below, but we could have as easily pointed it south. The important takeaway is that the orientation of the axis remains constant.
At the rightmost point, note that the spin axis of Earth points generally toward the Sun. This configuration happens in mid June, around June 21st. It is called the June Solstice. In the Northern Hemisphere this is the first day of summer. In the Southern Hemisphere it is the first day of winter.
When Earth is on the opposite side of the Sun, six months later, we see that Earth’s spin axis points generally away from the Sun. This happens in mid December, around December 21. This is called the December Solstice. In the north it is also called the Winter Solstice. In the south it is the Summer Solstice.
Both solstices represent the extreme extent of the Sun’s apparent motion above or below the equator. When the Sun is above the equator (north of the equator) it is summer in the north, winter in the south. When the Sun is below the equator (south of the equator) the seasons are reversed; it is summer in the south and winter in the north.
There are two positions that lie intermediate between the solstices. On these days, the spin axis of Earth points neither toward the Sun nor away from it. On these days the spin axis points in a direction perpendicular to the direction toward the Sun, and the Sun crosses the equator from one hemisphere to the other. These two days are given the special name Equinox.
There is one equinox in September and one in March; both are around the 21st of their respective months. On the September Equinox the Sun moves from the Northern Hemisphere to the Southern Hemisphere. On the March Equinox the Sun moves in the opposite direction, from south to north. Being midway between summer and winter, the Equinoxes are the first days of either spring or autumn, depending on which hemisphere is being considered.
The important idea to grasp from the images above is that the spin axis of Earth always points in the same direction. The northern axis is almost exactly lined up with the star Polaris, the “North Star.” There is no corresponding “South Star,” but the South Pole also points in a constant direction as Earth orbits the Sun.
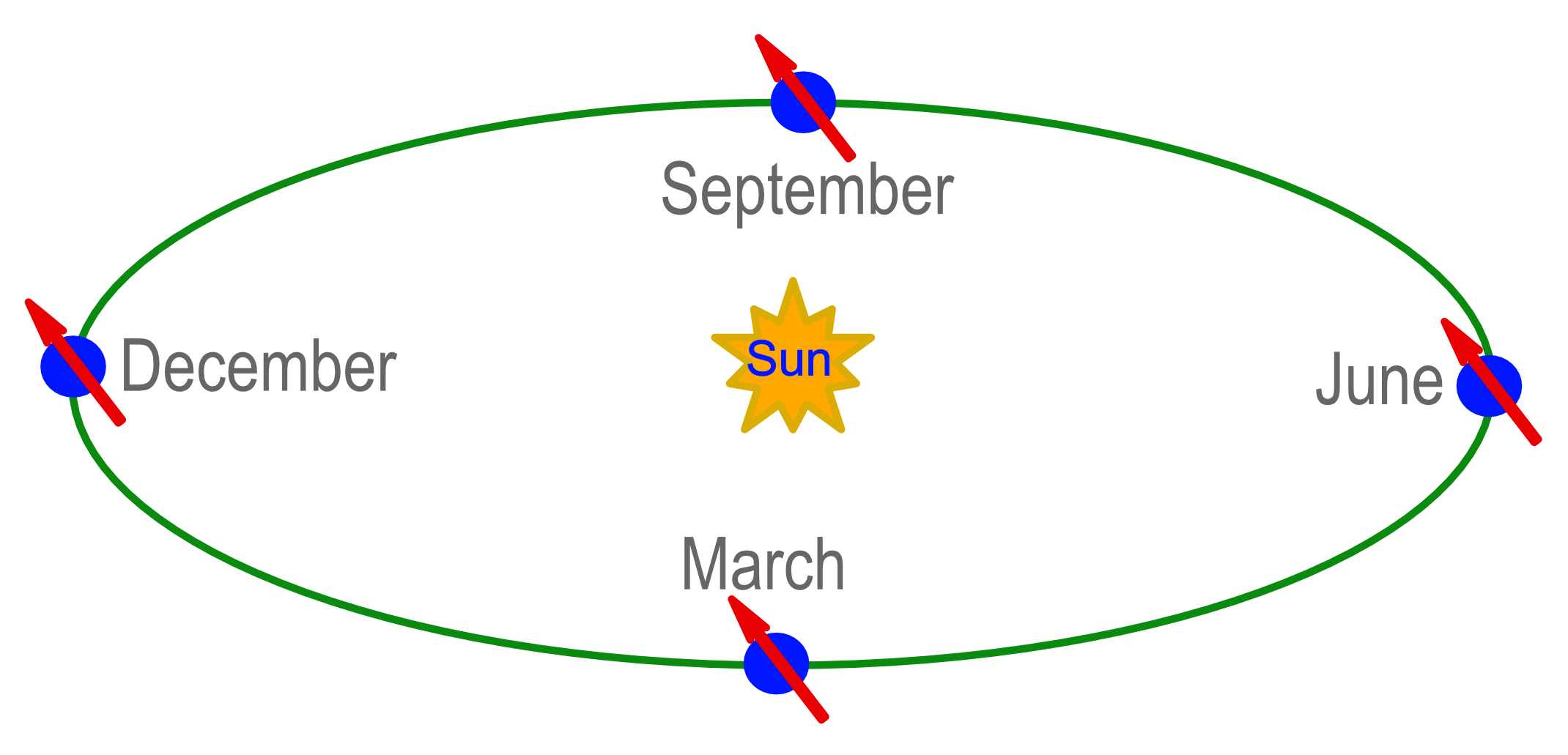
Just like Polaris, all the other stars remain in place throughout the year. But Earth doesn’t. It moves around the Sun, and its motion changes which stars we can see in the night sky. How can this be?
Have a look at the image below. It looks almost identical to the first one above. The only difference is that we have now added a few of the stars in the sky as reference points. These are the stars along the ecliptic plane (called the zodiac by astrologers) and are probably familiar to you, at least their names are probably familiar. While the positions of these constellations are approximately correct, each is shown as it appears to us looking up at it from Earth. They have not been transformed to fit into the perspective of the diagram. As a result, the star patterns near the bottom of the diagram (near Earth’s position in March) are flipped left-to-right (or east-to-west) from the what they should be.
So in short, you can use the positions of the Earth and constellations through the year to get an idea of what is visible at that time of year, but don’t use the diagram as an absolutely faithful representation of the real sky.
Remember, these stars remain essentially stationary as Earth orbits the Sun. That means that they are in the same place in June as they are in December, or at any other time of the year for that matter. It is only Earth that moves… E pur si muove!
Also, realize that the nighttime side of Earth, the dark side, is always the side pointing away from the Sun. Earth’s rotation carries us onto the dark side every night, and back onto the daylight (lit) side a few hours later. In the diagram we have shaded the nighttime side of Earth gray, just to make it clear which side is lit and which is dark. With this understanding in mind, can you tell which stars are visible at night in June? What about December? What about March and September?
If you said Scorpius/Sagittarius in June, Gemini/Taurus in December, Leo/Virgo in March and Pisces/Aquarius in September, you are correct. Of course, these constellations are visible at night at other times of the year, either in the early evening or early morning. But during the months listed they are high in the sky around midnight.
And just to make sure you are following along, which stars are visible during the day? If you said none, you are almost right. The Sun is the only star visible during the day, assuming there are not any clouds to block our view. All the other stars are made invisible by the brightness of the Sun. They are still there, of course, but the sky is too bright to see them. Only at dusk, when the Sun sets and the sky brightness begins to diminish, do the other stars begin to appear. First we see the very brightest. Then the fainter ones slowly come out as Earth’s rotation carries us farther onto the nighttime side and the Sun sinks farther below our horizon.
The important thing to notice is that the nighttime side of Earth points in different directions at different times of the year. In fact, it points in exactly the opposite direction in June than it does in December. This is also true for the parts of the sky visible during March and September nights, respectively. And we don’t have to wait three or six months to see this effect. It creeps along, a little every night. It is difficult - but possible - to notice the difference from one night to the next, but over a week or two it becomes very noticeable.
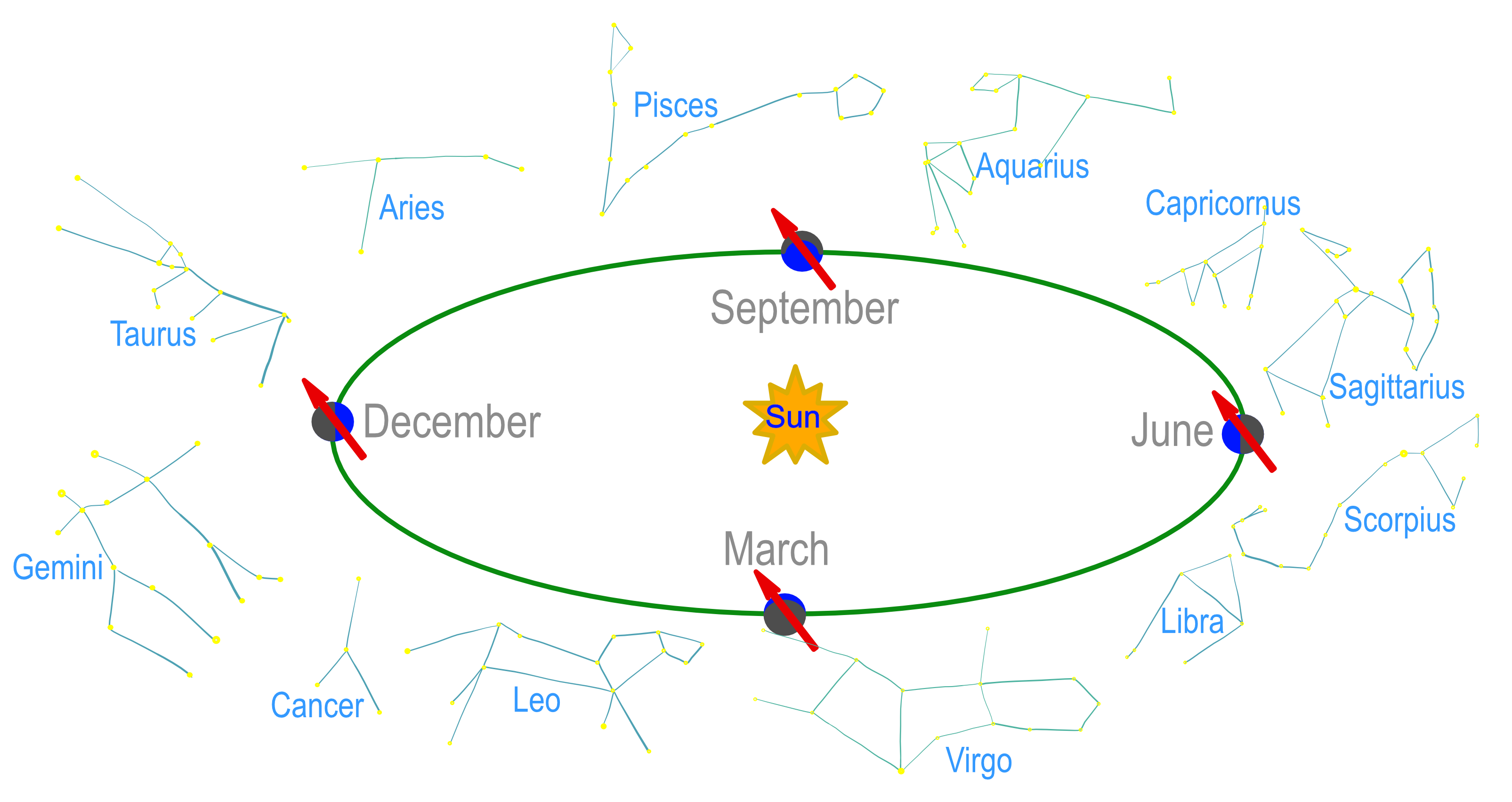
Apparent Motion of the Sun
The reason different parts of the sky are visible at different times of year is that the apparent position of the Sun varies throughout the year. Of course, the Sun’s position doesn’t really vary, the Earth’s position does. We can use our knowledge of Earth’s motion to understand how the Sun’s apparent position changes.
For purposes of argument, let’s imagine that it is local noon on June 21 at our position on Earth. Local noon means that the Sun is halfway between rising and setting. Astronomers call this position a transit. All objects in the sky transit once a day, just as they rise once a day and set once a day. The times of these risings, settings and transiting depend upon the object and our location on Earth. When the Sun is transiting, we call that local noon. It is different than the local noon for people at other locations (specifically, for people at different longitudes), just as the time of sunset and sunrise is different for locations with different longitudes. This is why we have timezones, 24 of them, circling around Earth.
Local noon is also different than the noon on your watch, unless you happen to be exactly in the middle of your timezone. It is the time of solar transit at your particular longitude on Earth.
We know that exactly one day later, it will be local noon again, and the Sun will again be transiting. That is the very definition of a day. This requires 24 hours to pass. But will the same stars be transiting as did the previous day? No. To understand why, look at the image below.
A Note About Time and Timezones
Everyone has their own local timezone to keep track of the position of the Sun for themselves. This is convenient most of the time. For times when it is not, and when a single common time base is useful, we all use a common standard called Universal Time (UT). This is basically the time in London, England. It is sometimes also called Greenwich Mean Time (GMT), and used to be called Zulu Time. Astronomers, pilots, navigators (and GPS devices) and others make constant use of UT. We won’t be bothered about these different time standards and will concentrate on local time (LT) because that is what is important for our view of the sky.
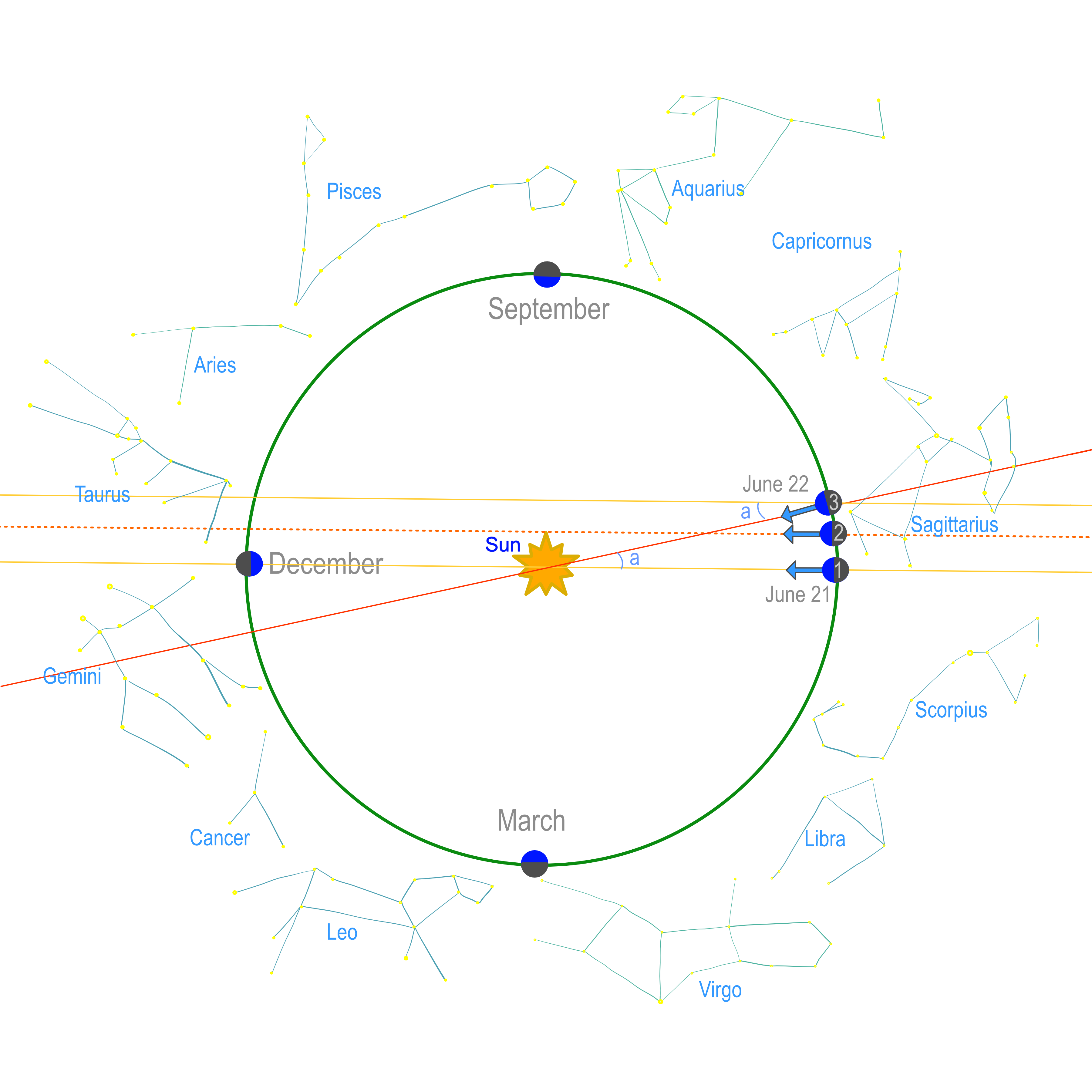
In this image we are again looking down on Earth’s orbit from a position directly above the Sun. As before, we see Earth located near the solstices and equinoxes. Only now it is shown at three different positions near the June solstice.
The first position (at bottom, labeled with a “1”) is on June 21. The time is noon for the location of the blue arrow. The arrow is taken to be at a fixed location on Earth’s surface and to rotate with it. We can tell it is noon because the arrow points in the direction of the Sun, so the Sun is transiting. This means it is halfway between rising and setting for a person at this position. Keep in mind that the Sun is only directly overhead for a person on the Tropic of Cancer, but it is transiting for anyone at the same longitude as the arrow.
The other two positions are for June 22. Both are roughly a day after the first position, though the diagram greatly distorts Earth’s motion for clarity’s sake. In the middle position, position 2, Earth has rotated through exactly 360 degrees, or one full rotation, since noon on June 21. We can tell this is the case because the blue arrows for this and the previous position are parallel. Note that in the second position, this is no longer the Sun’s location in the sky, and it is not quite local noon yet. The parallel (solid) yellow and (dotted) orange lines are provided as guides to make the directions of the arrows stand out more clearly.
Why is it not local noon yet in the second position? Because Earth has moved a small distance around the Sun as it orbits. In order to get to local noon, Earth must rotate through a small additional angle in order to make up for the small angle through which Earth has moved around the Sun.
In position 3, Earth has made up for its motion around the Sun, and it is local noon again. It has also moved a small additional distance around the Sun while making up ground, but this distance is greatly exaggerated in the diagram. It looks as though Earth has moved a long way around the Sun for this to happen, and thus taken a long time. But that is not the case. The diagram is only schematic. It shows the general phenomenon, but it is not completely accurate. Below we will deduce exactly how much extra angle, and thus extra time, is required for Earth to “catch up” to the Sun. We will find that the extra time required is very small.
Meanwhile, we have found is that there are basically two possible definitions for a “day.” One definition is the time between one solar transit and the next, local noon to local noon. This definition is given the name solar day. It is the day reflected on clocks, the one we use to keep track of time. The other definition is the time required between successive transits of the same star - other than the Sun.
Because stars (the vast majority of them) are so far away, the motion of Earth in its orbit does not affect the apparent direction to them. So in effect, the yellow and orange lines in the diagram all point to the same star in the sky because they are all parallel to each other; remember, parallel lines seem to meet at an infinite distance, and for our purposes the stars are infinitely far away. Thus, star transit to star transit corresponds to exactly 360 degrees of rotation. This is the definition of a sidereal day. Sidereal is a fancy word for star, and sidereal days are useful for keeping track of where given stars are on a given day. Most people never use them unless they are trying to navigate around the world or keep very accurate track of the time using the stars.
From our diagram it is clear that a sidereal day is shorter than a solar day. But by how much?
Note again the two parallel yellow lines that run from the Earth at positions 1 and 3. Because these lines are parallel we know that the indicated angles (labeled with “a”) made by running the red line through the Sun and Earth at position 3 are the same. They are called alternate interior angles, and we usually learn that they are equal in geometry in school. We have exaggerated the size of these angles greatly to make the diagram easier to read. We can compute their size to high accuracy using what we know about Earth’s motion, as we do now.
- Earth requires 365.26 days to orbit one time (360 degrees) around the Sun.
- Earth's orbit is nearly circular, so it moves at a near-constant speed around the Sun.

This means Earth moves 0.9856 degrees around the Sun in a day. As a result of this motion, it must spin through this extra angle for the Sun to transit again. We will simplify by setting the angle to exactly 1 degree. This is an approximation, but it is suitably good accuracy for our purpose. This is the value of the angles labeled \(a\) in the diagram.
It takes 24 hours for the Earth to rotate through one solar day. This is 1440 minutes. But 24 hours is noon to noon, or solar transit to solar transit. It corresponds to 361 degrees of rotation. This is the 360 degrees required for a star to transit, plus an additional degree for the Sun to transit. The time for the additional rotation can be found as below.

It takes roughly four minutes for Earth to rotate through one degree. Thus it takes Earth roughly four minutes to rotate through the additional degree to reach noon after it has rotated through 360 degrees. That means that stars seem to outpace the Sun by four minutes each day. They rise four minutes earlier, they transit four minutes earlier and they set four minutes earlier. Every day. Over a week this works out to nearly half an hour. Over a month, it means that the stars will be setting almost two hours earlier: stars setting (or rising) with the Sun during the first week of June will be setting (or rising) two hours before sunset (or sunrise) during the first week of July.
In this way, the night sky slowly changes over the course of the year, revealing different stars in different months. This is all dependent upon the location of Earth in its orbit. The table below provides a listing of where in the sky (which constellation) the Sun will be located for each month of the year. The table begins near the September equinox because that happens to be when this post is being written.
Sun Position Through The Year
Dates | Constellation |
---|---|
17 Sep - 30 Oct | Virgo |
30 Oct - 22 Nov | Libra |
22 Nov - 29 Nov | Scorpius |
29 Nov - 17 Dec | Ophiuchus |
17 Dec - 19 Jan | Sagittarius |
19 Jan - 15 Feb | Capricornus |
15 Feb - 11 Mar | Aquarius |
11 Mar - 18 Apr | Pisces |
18 Apr - 13 May | Aries |
13 May - 21 Jun | Taurus |
21 Jun - 20 Jul | Gemini |
20 Jul - 11 Aug | Cancer |
11 Aug - 17 Sep | Leo |
You might also note that in the table above, the Sun spends different amounts of time in different constellations. Why does this happen? It has to do with how the constellations are defined in the sky. Traditionally, the definitions were not very precise. But the official constellations today are defined by the International Astronomical Union (IAU) to cover all parts of the sky very precisely. It is similar to the way the boundaries of countries cover every part of a continent, with no gaps being left. The areas where these constellations intersect the ecliptic are not even, and so the Sun spends different amounts of time in each.
You might also be surprised that there is an “extra” constellation in the table; there are 13 listed, not twelve. Where did Ophiuchus come from? That constellation is not part of the astrological zodiac at all. Again, this is to do with the precession of the equinoxes and the hard boundaries set by the IAU. With the current orientation of the equinoxes, the Sun now spends time in what is officially called Ophiuchus. But if you happen to be born in the first part of December, you will not be an “Ophiuchan” according to astrologers. As we have already discussed, they do not even recognize that precession has changed the apparent location of the Sun in the past 2000 years. Certainly they will not concern themselves with the arbitrary definitions by a different set of skywatchers regarding the boundaries between constellations.
The images below show where the Sun is located in the sky as observed from Earth for March 21, June 21, September 21 and December 21. These are the equinoxes, when the Sun is on the equator, and the solstices, when the Sun is at its greatest extent north and south of the equator. The images were created using SkySafari Pro ver. 6.5.0. Click on the images to view a larger version of them, and then use the Back button on your browser to return to this page.
In these images and table we are given the region of the sky toward the Sun (the daylight side). That means the part of the sky visible at night is in the opposite direction. So the stars behind the Sun in the June are visible to us at night in December, the stars behind the Sun in March are visible at night in September, and so on. The images depict local noon, so this would be the sky seen near midnight six months later or earlier.
Finally, we have shown only the parts of the sky close to the ecliptic, and we have only marked out the constellations that fall along it. We did this to keep things simple. But bear in mind that if these stars are visible at night, then the stars around them are also visible. If these stars are close to the Sun, and thus up during daylight hours, then nearby stars are too.
Precession of the Equinoxes
Those of you familiar with the astrological zodiac will probably notice that the dates here are not the same as the dates used for “birth signs.” This is because the table shown here provides the actual position of the Sun for the time this is being written, in 2020. The astrological system was set up at a very different time. Astronomers say it uses a different epoch. It reflects where the Sun was roughly 2000 years ago, when the current astrological system was set up. But why are the two different?
If you think it has something to do with the motion of Earth, you are right! In our discussion above we left off some of Earth’s motions because they were not relevant to that discussion. They are not noticeable over the course of a year, or even many years. However, over longer periods of time these motions do become noticeable. One of these is called precession.
Precession in the slow drift of Earth’s rotation axis. It is the same sort of motion that is familiar from watching a top spin: as the top goes round and round, its spin axis also slowly traces a small circle around the vertical direction. The precession period is always much longer than its spin period. The spin orientation of Earth also slowly traces a small circle in the sky, and just like a top, the time required to complete one circuit of precession is much longer than the time required for Earth to spin one time on its axis.
Precession has several affects on how the sky looks from Earth. First, the North and South Poles gradually shift the direction they are pointing, and their projections (the North and South Celestial Poles) carve out a circle on the sky. The celestial North and South Poles complete their path around this circle once every 26,000 years, roughly. So this means that, in another thousand years, the North Star, Polaris, will be quite some distance from the celestial North Pole. It will not return to its current spot, nearly coincident with the pole, for another 25,000 years after that.
You can use the four tabs below to see where the North Celestial Pole is located at a few selected times in the past and future.
1000 AD
A view looking north toward the north celestial pole (marked NCP) in the year 1000 AD. Note that the star Polaris (the North Star) was not aligned with the pole at that time.
Click on the image for a larger view. Image generated by SkySafari Pro.
2000 AD
A view looking north toward the north celestial pole (marked NCP) in the year 2000 AD. Note that the star Polaris (the North Star) was aligned closely with the pole at that time. This alignment will continue for many years, but Polaris will eventually move off the pole as Earth’s precession directs the pole to a different part of the sky.
Click on the image for a larger view. Image generated by SkySafari Pro.
3000 AD
A view looking north toward the north celestial pole (marked NCP) in the year 3000 AD. Note that the star Polaris (the North Star) will no longer be aligned with the pole at that time.
Eventually precession will reestablish the alignment, but that will not happen for 25,000 years.
Click on the image for a larger view. Image generated by SkySafari Pro.
The other effect of Earth’s precession is more subtle. Since the spin axis of Earth is tilted 23 degrees with respect to its orbital plane, there are two points at which the planes intersect. These two points are the spots where the Sun is located on the equinoxes. The Sun is always in the plane of Earth’s orbit, so the only two times it can be directly above the equator are when it is at the points where the equatorial plane intersects the ecliptic plane. To make things even more confusing, these points are also called equinoxes. So “equinox” can refer to a day of the year or a place in the sky. The context will generally make it clear which meaning is the appropriate one.
If you imagine a line connecting the two equinoxes and running through the center of Earth, you can probably see that this line will be perpendicular to Earth’s spin axis. The animation above might help you visualize the geometry. It shows the ecliptic plane, represented by the green circle, and the equatorial plane, represented by the yellow circle - it appears as an ellipse here because it is tilted, and so it is foreshortened along the line of sight for the same reason as we discussed in the first section of this post. The earth is the blue disk at the center, and its spin axis is represented by the yellow arrow, which points to the North Celestial Pole. You can click on the image to open a larger version in a new window.
As the spin axis slowly precesses, the line through the equinoxes - it is called the line of nodes - must follow it. This is because the equatorial plane is always perpendicular to the spin axis, so it has to rotate around at the same rate the spin axis does. And of course, if the equatorial plane is rotating, then its intersection with the ecliptic must be rotating, too. The effect is illustrated in the animation.
Here’s the thing though: the stars do not move while this is happening. Even on timescales of 26,000 years their intrinsic motion is pretty much negligible. So the line of nodes sweeps out a path in the sky in sync with the poles. At the moment, the line of nodes points to Pisces in one direction, and to Virgo in the other. But a couple thousand years ago, it pointed to Aries on one side and Libra on the other. A thousand years from now, precession will have rotated the line of nodes so that that it will pass through Aquarius and Leo.
You might have heard at some point of “The Age of Aquarius.” Now you know what is refers to: the time when the (northern) Spring Equinox is pointing toward Aquarius.
Since the seasons are determined by the positions of the equinoxes (where the Sun moves from the Southern to Northern Hemisphere, or the opposite) and solstices, the position of the Sun in a given season also slowly changes. This change will not be enough to notice over a lifetime, or even many lifetimes. But even year to year it can be noticeable if you are trying to do something that depends to high precision on the positions of the stars. Keeping time and pointing a telescope are two examples of tasks that depend on knowing the exact epoch, i.e., the exact position of the equinoxes in the sky.